Industry News: Microvascular Function: Experimental & Computational
Introduction
Microvascular function is important for ensuring tissue perfusion and generally the health of the vasculature. The microvessels include arterioles, capillaries, and venules and play a vital role in facilitating nutrient exchange, removing waste, and blood flow regulation at tissue level. Such diseases, including diabetes, cardiovascular diseases, neurodegenerative disorders, and many others, have been associated with dysfunction in this system; thus, it is an important system in the process of disease progression and outcome.
There has been a rising movement in recent years to support the use of computational and experimental techniques in the study of microvascular function. By incorporating such methodologies into the study, it is beneficial to gain understanding of the structural and functional elements of microvessels and to evaluate their function. This approach could help predict disease conditions as well as the response to therapy, thereby leading to improved clinical outcomes.
Industrial Applications of Microvascular Studies
Pharmaceutical Development
Microvasculature studies are critically important in drug discovery, particularly with cardiovascular and cancer therapies. These studies could potentially help in the evaluation of drug effects in the microvasculature, which is a network of tiny blood vessels that influences how tissue perfusion and drug delivery occur [1] [2]. Thus, cardiovascular disease research utilises the function of micro vessels in drug development related to therapeutic strategies to enhance blood flow and reduce inflammation. Computational models used in the cardiovascular field are computational fluid dynamics (CFD), structural or finite element analyses (FEA), and fluid-structure interaction (FSI) models [2].
Computational fluid dynamics is applied for calculating fluid flow patterns from inside the heart to the vessels. The finite element analysis models analyze the structural behavior of the cardiovascular system in terms of stress and deformation. Fluid-structure interaction approaches consider effects from flow and structural parts.
In cancer treatment, potential drug delivery and efficacy mainly depend on the behavior of the tumor’s microvasculature, such as abnormal vessel growth and permeability [1]. Through these predicted responses, microvascular models improve the treatment design so that it can target tumors more effectively.
In oncology, anti-angiogenic therapies that target the tumor vascular demonstrate potential promise in preventing the growth of the tumors along with enhancing the delivery of drugs [6]. Therefore, it helps in optimization of formulations that enhance vascular health in both fields with microvascular function.
Personalized Medicine
Advances in computational models of microvascular function are revolutionizing personalized medicine through the provision of customized treatment for differing patients. In this respect, such models help in simulating individual variability in microvascular function, which could influence drug absorption and disposition. This further allows clinicians to design tailor-made therapies suitable to the case of each patient [1]. For example, PDOs (patient-derived organoids) and (organ-on-chip) OOC can recreate patient-specific microvascular environments; hence, they supply exclusive information on drug-specific responses that are in accordance with the patient’s physiological state.
Patient-derived organoids
PDO cultures have also been found in malignant tissues such as biliary, pancreatic, and breast. Additionally, PDOs that mimic metastasis have been established in various settings, for instance, colorectal peritoneal masses. However, these PDO models are suitable for multi-organ-system etiologies [3]. These innovations have improved the effectiveness of personalized treatment regimes in oncology and cardiovascular care to a great extent.
Organ-in-Chip
In more complex disease systems such as those mentioned, organ-in-chip acts as a system for using the necessary components of a good model: scale, personalization, and mimicry. OIC models include fluid motion and tissue types to grow multiple organoids in sync [3]. A dynamic system like Organ-in-the-Chip is utilized for etiology research in multi-organ pathologies like inflammatory bowel disease (IBD) and testing pharmacokinetics such as MDR1 efflux effects in the intestine [5] [1].  In addition, the integrity of lumen and drug diffusion in (Organ-in-chip) OIC is used to evaluate the efficacy of drugs.
Machine learning Algorithms
Computational models integrate several sources of biological data, such as genomics and imaging, to simulate the functionalities of micro vessels altering drug responses or the progression of disease. For instance, through machine learning algorithms, predictions can be made for individual patient outcomes depending on patients’ characteristic differences in microvascular function [7]. This, consequently, promotes more precision in cardiovascular and cancer therapies. However, such models have several challenges associated with them concerning noise in data, validation, and ethical applications to heterogeneous populations.
Medical Devices and Diagnostics
The development of novel medical devices and diagnostic tools is significantly influenced by the microvascular research field. Novel technologies, such as microfluidic devices, are used to measure microvascular health, and improvements in imaging methods help to visualize real-time microvascular function. These advancements are critical in diagnosing conditions related to microvascular abnormal activity, such as ischemic heart disease and cancer development [1]. Devices that can monitor microvascular blood flow or detect early signs of endothelial dysfunction would improve early diagnosis and treatment options.
These devices are also used in detecting microvascular obstruction post-myocardial infarction and are critical in evaluating patient prognosis [8]. Furthermore, emerging diagnostic imaging technologies, such as cardiac magnetic resonance imaging (MRI), provide detailed real-time assessments of microvascular function and help in earlier detection of diseases such as CMD and hypertrophic cardiomyopathy.
Conclusion
In a nutshell, microvascular function is an important element in a wide range of biomedical research and industry applications, especially in the development of pharmaceuticals, individualized medicine, and production of advanced medical devices. Thus, microvascular research is important for the discovery of drugs involved in the treatment for cardiovascular and cancer diseases, improvement of drug efficacy, and optimization of formulations. Personalized medicine and real-time imaging technologies have transformed clinical practice by providing better insights into microvascular dysfunction. Together, these advances point to how valuable it will be to understand and harness microvascular function in multiple dimensions of healthcare and drug development.
References
- Zitter, R., Chugh, R.M. and Saha, S., 2022. Patient Derived Ex-Vivo Cancer Models in Drug Development, Personalized Medicine, and Radiotherapy. Cancers, 14(12), p.3006.
- Stefanini, G., Luraghi, G., Sturla, M. and Migliavacca, F. (2023) Use of computer models in cardiovascular therapy to advance precision medicine.
- Narasimhan, V.; Wright, J.A.; Churchill, M.; Wang, T.; Rosati, R.; Lannagan, T.R.M.; Vrbanac, L.; Richardson, A.B.; Kobayashi, H.; Price, T.; et al. (2020) Medium-throughput Drug Screening of Patient-derived Organoids from Colorectal Peritoneal Metastases to Direct Personalized Therapy. Clin. Cancer Res 26, 3662–3670.
- Mittal, R.; Woo, F.W.; Castro, C.S.; Cohen, M.A.; Karanxha, J.; Mittal, J.; Chhibber, T.; Jhaveri, V.M. (2019) Organ-on-chip models: Implications in drug discovery and clinical applications. J. Cell. Physiol. 234, pp. 8352–8380.
- Kasendra, M.; Luc, R.; Yin, J.; Manatakis, D.V.; Kulkarni, G.; Lucchesi, C.; Sliz, J.; Apostolou, A.; Sunuwar, L.; Obrigewitch, J.; et al. (2020) Duodenum Intestine-Chip for preclinical drug assessment in a human relevant model. eLife , 9, e50135.
- Marano, P., Wei, J. and Merz, C.N.B. (2023) Coronary microvascular dysfunction: what clinicians and investigators should know. Current Atherosclerosis Reports, 25(8), pp.435-446.
- Fröhlich, H., Balling, R., Beerenwinkel, N., Kohlbacher, O., Kumar, S., Lengauer, T., Maathuis, M.H., Moreau, Y., Murphy, S.A., Przytycka, T.M. and Rebhan, M. (2018) From hype to reality: data science enabling personalized medicine. BMC medicine, 16, pp.1-15.
- Marano, P., Wei, J. and Merz, C.N.B. (2023) Coronary microvascular dysfunction: what clinicians and investigators should know. Current Atherosclerosis Reports, 25(8), pp.435-446.
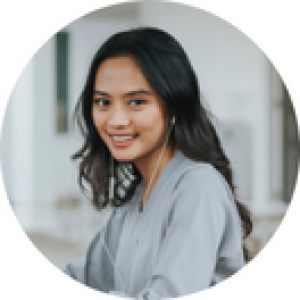
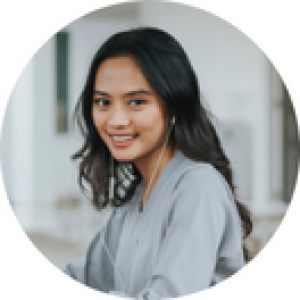