Study Guide On Microvascular Function
Introduction to Microvascular Function
Microvascular function is a term that refers to the roles and activities of small vessels, such as capillaries, arterioles, and venules, within the circulatory system. The vessels allow for the performance of obligatory processes, like nutrient and gas exchange, regulation of blood flow, and modulation of immunity, all of which are important to homeostasis [1]. Under normal physiological conditions, when there is increased myocardial oxygen, this results in heightened levels of metabolic substances. Consequently, the high levels of metabolic substances promote small arteriole dilatation. As a result, this tends to lower the pressure within the lumen of the medium arteriole, increase blood flow, dilate large arterioles, and therefore increase myocardial perfusion. Furthermore, coronary microvascular endothelial cells (CMECs) play a crucial role in ensuring normal function of the coronary microvasculature. This microvascular function study guide introduces the endothelial cell’s crucial roles within the small blood vessels and their mechanisms in keeping cardiovascular health, with emphasis on physiological processes.
Physiological Role of Microvascular Function
-
Blood Flow Regulation
The microvascular system plays an important role in coordinating blood flow through actions such as vasodilation and vasoconstriction. These may involve the release of vasoactive substances such as nitric oxide from the endothelial cells into the lumen, which causes relaxation of the smooth muscle cells in the vessel walls and subsequent enhancement of blood flow [2]. Most of the resistance to coronary blood flow and its control occurs in microcirculation [2]. This modulation of microcirculatory resistance keeps the coronary blood flow and myocardial oxygen consumption high enough for the functioning of the heart in almost all conditions.
-
Nutrient and Gas Exchange
The blood capillaries are the smallest vessels involved primarily in the exchange of oxygen, carbon dioxide, nutrients, and waste products with tissues [4]. The thin walls of these capillaries allow for efficient diffusion, which is necessary for cellular metabolism.
-
Inflammation and Immune Response
Microvascular function plays an important role in inflammation and immune responses. Following tissue injury or infection, adhesion molecules expressed on endothelial cells facilitate leukocyte adhesion and transmigration, allowing for immunity within the damaged areas [3].
Experimental Approaches to Study Microvascular Function
-
In Vivo Techniques
In vivo techniques, innovations like intravital microscopy and laser Doppler flowmetry offer real-time microvascular dynamics [5]. Thus, blood flow and vascular responses can be viewed under various physiological conditions.
-
In Vitro Techniques
In vitro methodologies, organ perfusion systems, and endothelial cell culture assays are used to make controlled experiments on microvascular function possible [4]. In addition, capillary permeability assays further help explain the mechanisms governing nutrient exchange and barrier function.
-
Molecular and Cellular Techniques
Gene expression and protein assays (ELISA, Western blot, etc.) will help identify which signaling pathways may be contributing to the effects of microvascular dysfunction [6]. Flow cytometry is applied to studies examining the activation state of leukocytes in inflammatory responses.
Computational Models of Microvascular Function
-
Overview of Computational Approaches
Computational modeling has emerged to be a very powerful tool in the research world of microvessels. It facilitates a deeper understanding of hemodynamics, permeability, and overall microvascular behavior under varying conditions.
-
Mathematical Models
Poiseuille’s law and capillary filtration theory are important mathematical models helping predict blood flow and fluid exchange across the capillaries [7]. These models enable quantification of microvascular function and dysfunction. These mathematical models explain the behavior of Circulating tumour cells (CTCs) through blood vessels with the flow through capillaries and the function of erythrocytes.
-
Simulation Software
COMSOL Multiphysics and ANSYS are the most widely used software tools for computational fluid dynamics (CFD) simulation of microvascular blood flow [8]. Such simulations are used to effectively illustrate a great deal about blood flow patterns and shear stress distributions within a microvascular network.
-
Integrative Models
Integration with experimental data with computational models may enhance understanding of microvascular function [9]. Models that bridge the gap between cellular and systemic levels offer a complete understanding of the microvascular response in health and disease. Through integration of various emerging diagnostic techniques into healthcare systems, we can envision a transformative shift in detecting and managing microvascular complications.
Pathophysiological Implications of Microvascular Dysfunction
-
Common Diseases Associated with Microvascular Dysfunction
Microvascular dysfunction has been demonstrated to be associated with a variety of diseases, including diabetes, hypertension, and atherosclerosis [10]. These latter conditions typically result in decreased blood flow and thus tissue hypoxia, contributing to organ damage.
-
Experimental Evidence
Clinical studies and animal models demonstrate that microvascular dysfunction serves as a precursor to many chronic diseases. For example, diabetes-induced microvascular changes can result in retinopathy, nephropathy, and neuropathy [11]. Even though several experimental studies show coherence between sublingual and other organ surfaces’ microcirculation, clinical circumstances may cause differences in behavior between different surfaces’ microcirculatory beds.
-
Computational Insights
Most importantly, computational modeling has provided greater insight into mechanisms causing microvascular dysfunction [4]. For example, in diabetic models, altered blood flow dynamics and endothelial permeability have been determined as potential therapeutic targets. Further, advanced modeling approaches, coupled with innovative software tools and new computational modeling methods, can be used to link knowledge with machine learning-based predictions made from multi-scale data.
Emerging Trends and Future Directions
-
Technological Advancements
Recent advances in imaging techniques, including high-resolution ultrasound and magnetic resonance imaging (MRI), have improved our capability for the non-invasive assessment of microvascular function [11]. These technologies permit early detection of microvascular complications.
-
Personalized Medicine and Microvascular Function
The integration of microvascular assessments into personalized medicine approaches has been increasing in numbers recently. It helps in identifying individual microvascular health profiles that can guide targeted interventions and thus improve patient outcomes. Further, it is observed that the research on personalized medicine aims to transition from system analysis to system control and helps to plan optimal medical interventions [9]. Personalized medicine utilizing novel computational modeling approaches can go beyond the current machine learning methodology to enhance personalization.
-
Research Opportunities
Future studies should fill the gaps in our knowledge about microvascular function, using the emerging diseases as well as therapeutic modalities. Experimental and computational models may be applied through inter-disciplinary approaches to increase our ability to deal with the vascular complexity. The future models could help physicians to zoom and filter specific aspects of the model based on requirement [9]. Further, these models can help physicians to understand disease development over time. However, developing such systems is greatly helpful for scientists and physicians to communicate between them and make complex machine learning models more interpretable.
Conclusion
Understanding the mechanisms by which microvascular function maintains physiological homeostasis has immense implications for health and disease. Experimental and computational approaches are critical advancements toward further understanding these mechanisms and addressing the challenges associated with microvascular dysfunction. This study guide is an excellent accompaniment for readers, be they physicians or researchers, in several ways. Further, it bridges the gap between theoretical understanding and practical application, making it an all-round source for both clinicians looking to enhance patient care and researchers looking to open new frontiers in vascular biology.
References
- Yang, Z., Liu, Y., Li, Z., Feng, S., Lin, S., Ge, Z., Fan, Y., Wang, Y., Wang, X. and Mao, J. (2023) Coronary microvascular dysfunction and cardiovascular disease: Pathogenesis, associations and treatment strategies. Biomedicine & Pharmacotherapy, 164, p.115011.
- Marano, P., Wei, J. and Merz, C.N.B., 2023. Coronary microvascular dysfunction: what clinicians and investigators should know. Current Atherosclerosis Reports, 25(8), pp.435-446.
- Rizzoni, D., De Ciuceis, C., Szczepaniak, P., Paradis, P., Schiffrin, E.L. and Guzik, T.J., 2022. Immune system and microvascular remodeling in humans. Hypertension, 79(4), pp.691-705.
- Apeldoorn, C., Safaei, S., Paton, J. and Maso Talou, G.D., (2023) Computational models for generating microvascular structures: Investigations beyond medical imaging resolution. WIREs Mechanisms of Disease, 15(1), p.e1579.
- Coccarelli, A. and Nelson, M.D. (2023) Modeling reactive hyperemia to Better Understand and assess microvascular function: a review of techniques. Annals of Biomedical Engineering, 51(3), pp.479-492.
- Frenette, A.P., Rodríguez-Ramos, T., Zanuzzo, F., Ramsay, D., Semple, S.L., Soullière, C., Rodríguez-Cornejo, T., Heath, G., McKenzie, E., Iwanczyk, J. and Bruder, M. (2023) Expression of Interleukin-1β protein in vitro, ex vivo and in vivo salmonid models. Developmental & Comparative Immunology, 147, p.104767.
- Rajput, S., Sharma, P.K. and Malviya, R. (2023) Fluid mechanics in circulating tumour cells: role in metastasis and treatment strategies. Medicine in Drug Discovery, 18, p.100158.
- Zhou, L., Elemam, M.A., Agarwal, R.K. and Shi, W. (2024) Computational Fluid Dynamic (CFD). In Discrete Element Method for Multiphase Flows with Biogenic Particles: Agriculture Applications (pp. 65-82). Cham: Springer Nature Switzerland.
- Fröhlich, H., Balling, R., Beerenwinkel, N., Kohlbacher, O., Kumar, S., Lengauer, T., Maathuis, M.H., Moreau, Y., Murphy, S.A., Przytycka, T.M. and Rebhan, M. (2018) From hype to reality: data science enabling personalized medicine. BMC medicine, 16, pp.1-15.
- Rehan, R., Yong, A., Ng, M., Weaver, J. and Puranik, R. )2023) Coronary microvascular dysfunction: a review of recent progress and clinical implications. Frontiers in Cardiovascular Medicine, 10, p.1111721.
- Raut, S.S., Acharya, S., Deolikar, V. and Mahajan, S. (2024) Navigating the frontier: emerging techniques for detecting microvascular complications in type 2 diabetes mellitus: a comprehensive review. Cureus, 16(1).
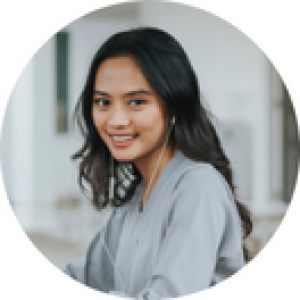
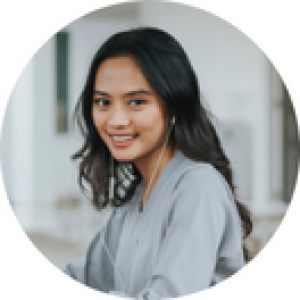