Future of Microvascular Research: Experimental and Computational Advances
Introduction
The microcirculation is constantly changing as people grow and adapt, both in health and sickness, while maintaining tissue health and homeostasis. Microcirculation is the ‘end point’ of the circulatory system, which consists of a network of microvessels that transport oxygen and nutrients, meanwhile eliminating waste items from organ tissues. Microcirculation helps blood transport and intercellular signaling in response to local demands, as well as redistributing hydraulic loads and promoting inflammatory processes [1]. Recent advancements on both experimental techniques and computational modeling open new avenues for the understanding of microvascular physiology and pathology by developing mathematical modeling. This article will explore more on emerging trends and technologies on microvascular functions.
Background and Significance
Microvascular dysfunction is defined as a structural and functional remodeling of the microcirculation resulting in disturbance of autoregulation of blood flow due either to dysfunctional coronary vasodilator capacity or increased reactivity to microvascular vasoconstriction [2]. Microvascular dysfunction can result in various diseases such as diabetes, hypertension, and cardiovascular disorders. Structural as well as functional abnormalities in these blood vessels result in endothelial dysfunction and abnormalities within the myocardium with its effects on the intramural microvasculature [2]. To address the developing issues related to microvascular dysfunction and other microvascular complications such as Type 2 Diabetes Mellitus, understanding the function of microvascular is vital. It helps to develop effective treatment or therapeutic strategies. Despite the traditional methods such as animal model and histological validations, it is essential to discover new innovative strategies to overcome limitations in these traditional methods [3] [4].
However, some of the latest technological innovations, such as advanced imaging techniques, microfluidics, and computational modeling, have potentially enhanced our understanding related to microvascular dynamics. Employing mathematical models can accelerate the physiological understanding the underlying issues and help in finding quantities, which is difficult in experimental model [1]. Thus, it will be possible to use both experimental and computational approaches, which will provide a better understanding of microvascular dynamics to pave the way for innovative therapies and diagnostics.
Current State of Microvascular Research
The traditional approaches in the study of microvascular function mainly rely on imaging and invasive techniques. Unfortunately, such techniques usually lack details of microvascular dynamics. The use of non-invasive imaging techniques, including computed tomography (CT), magnetic resonance imaging (MRI), and positron emission tomography (PET), has increased resolution but cannot be used for widespread application because the technical costs of acquisition and exposure to radiation are too high.
The study of microvascular function is at a very crossroad, since common existing imaging and intrusive techniques fail to provide a complete dynamic of microvasculature. Non-invasive techniques such as CT, MRI, and PET are promising but limited by high costs along with radiation exposure, making it hard for broad applications. Pubrica offers research services encompassing advanced data analysis, computational modeling, and manuscript support, enabling you to take your microvascular research to new heights and overcome such challenges.
Emerging Trends and Technologies
High-Resolution Imaging
Newer imaging techniques such asmultiphoton microscopy (two-photon microscopy) and optical coherence tomography (OCT) help the researchers to visualize microvascular structures and functions in high precision. OCT provides detailed retina images that help to detect subtle changes in retinal thickness and morphology. It is widely used in clinical practices in detecting and monitoring DR (diabetic retinopathy) and DME (diabetic macular edema) [5]. On the other hand, microvascular morphological properties such as diameter, segment length, and tortuosity can be extracted using a two-photon microscopic technique employing a deep-learning algorithm [6]. In addition, non-invasive techniques such as computed tomography (CT), magnetic resonance imaging (MRI) and positron emission tomography (PET) are also used in evaluating microvascular function.
Microfluidics
This technique helps to develop more physiologically relevant blood vessel models to produce continuously perfused, multi-cellular, long-term cultures to overcome the limitations of 2D models. Further, this microfluidic system utilizes sub-millimeter-sized channels to accurately control extremely small amounts of fluid in the order of 10−9 to 10−18 [7]. Further, as an ideal platform, microfluidics technology helps in bridging the gap between simple, low-cost 2D in vitro models and complex, expensive in vivo models [8]. It helps in developing high-throughput biological modeling of microvasculature by reducing the consumption of expensive reagents and precious cells.
In the development of microfluidic systems, organ-on-a-chip models are one of the significant innovations that replicate the microenvironment of the microvasculature, allowing for more precise disease modeling and drug testing [9]. Further, these models have specifically proven valuable in investigating complex diseases like diabetes and cancer caused by microvascular dysfunction.
3D Bioprinting of Vascular Networks
Improvements in 3D bioprinting technology have enabled the creation of bioengineered tissues with intrinsically integrated functional microvascular networks [9]. These technologies can create innovation in tissue engineering and regenerative medicine, leading to new ways to treat cardiovascular disease.
Omics Technologies
Currently, the availability of single-cell RNA sequencing and multi-omics techniques enables the researchers to tackle a much greater level of detail for microvascular heterogeneity. This allows us to understand how microvascular function varies by tissue type and disease state [10]. Further, the emerging methodologies in genomic and proteomic analyses provide promising opportunities for early detection of microvascular complications in patients with type 2 Diabetes mellitus [11]. However, further research is essential to address challenges, and refined methodologies can produce effective results in clinical settings.
Computational Modeling
Computer modeling can be a useful adjunct to hypothesis testing, making predictions and providing quantitative insight into vascular regulation dynamics that cannot be accessed directly during in vivo experimentation [1]. Computational modeling helps the researchers to investigate the mechanics of the delicate system while minimizing using animals in experiments [9]. The research simulates microvascular flow dynamics by using advanced computational techniques, such as finite element analysis and agent-based modeling, to forecast the responses such systems would exhibit to pathological conditions.
In a study, Solovyev et al. (2013) defined a hybrid model, combining a lumped model for blood flow with an agent-based model of skin injury, to evaluate the role of blood flow on the development of pressure ulcers in spinal-cord injury patients [1]. Complex constitutive models can be used to determine hemodynamic features and wall properties of vessel reflecting physiological and pathological conditions like aging or hypertension. Similarly, blood flow variables can be computed using numerical analysis techniques such as finite differences, finite elements, and finite volumes [1]. It can be extended to large vessel networks by imposing mass and momentum conservation.
Machine Learning and AI
The integration of machine learning and artificial intelligence (AI) into microvascular research can pave the way for innovation in predictive modeling. AI helps in analyzing patterns in large volumes of datasets, which provide a clear understanding of microvascular behavior under different physiological conditions.
Novel Therapeutic Approaches
Therapeutic strategies that are targeted at microvasculature, such as by using angiogenesis inhibitors or pro-angiogenic therapies, may open new horizons in the treatment of diseases associated with dysfunctional microvascular function [12]. Similarly, the use of microvascular biomarkers does hold promise for advancing personalized medicine.
Future Directions
S.NO | Future Direction | Scope | References |
1 | Development of Biomimetic Microvascular Models | Establish models of human microvascular behaviour using in vitro microfluidic systems. | [13] |
2 | Integration of AI in Computational Modeling | Utilize machine learning algorithms to predict microvascular responses based on large datasets. Further, integrating nanotechnology and AI into microvascular leads to the development of more sophisticated models capable of predicting microvascular function in real-time. | [12] |
3 | Personalised medicine | By leveraging computational models and experimental data, researchers can create individualized treatment plans that optimize microvascular function for each patient | [1] |
4 | Enhanced Biomarker Discovery | Use omics technologies to identify new biomarkers of microvascular dysfunction. Further, developing advanced technologies enable researchers to get clear understanding of microvascular complications. | [5] |
Conclusion
The emergence of new trends in experimental and computational approaches is rapidly evolving towards the understanding of the function of microvessels. Combining these methodologies will make possible to the development of novel therapies and improvement in the clinical management of diseases involving dysfunction of the microvascular system. As this field progresses, interdisciplinary collaboration will be useful in unlocking the full potential of these new technologies.
References
- Coccarelli, A. and Nelson, M.D. (2023) Modeling reactive hyperemia to Better Understand and assess microvascular function: a review of techniques. Annals of Biomedical Engineering, 51(3), pp.479-492.
- Rehan, R., Yong, A., Ng, M., Weaver, J. and Puranik, R. (2023) Coronary microvascular dysfunction: a review of recent progress and clinical implications. Frontiers in Cardiovascular Medicine, 10, p.1111721.
- Cifuentes-Mendiola, S.E., Solís-Suarez, D.L., Martínez-Davalos, A. and García-Hernández, A.L. (2023) Macrovascular and microvascular type 2 diabetes complications are interrelated in a mouse model. Journal of Diabetes and its Complications, 37(5), p.108455.
- Liu, D., Lin, R., Tao, B., Hu, J., Cheng, L., Lou, X., Li, M., Li, S., Zhu, Y., Li, N. and Fang, Y. (2023) Translational large animal model of coronary microvascular embolism: characterization by serial cardiac magnetic resonance and histopathology. The International Journal of Cardiovascular Imaging, 39(9), pp.1741-1752.
- Raut, S.S., Acharya, S., Deolikar, V. and Mahajan, S., 2024. Navigating the frontier: emerging techniques for detecting microvascular complications in type 2 diabetes mellitus: a comprehensive review. Cureus, 16(1).
- Li, Baoqiang, Andriy Yabluchanskiy, Stefano Tarantini, Srinivasa Rao Allu, Ikbal Şencan-Eğilmez, Ji Leng, Mohammed Ali H. Alfadhel et al. (2023) “Measurements of cerebral microvascular blood flow, oxygenation, and morphology in a mouse model of whole-brain irradiation-induced cognitive impairment by two-photon microscopy and optical coherence tomography: evidence for microvascular injury in the cerebral white matter.” Geroscience 45, no. 3 : pp:1491-1510.
- Bathrinarayanan, P.V., Hallam, S.M., Grover, L.M., Vigolo, D. and Simmons, M.J.H. (2024) Microfluidics as a Powerful Tool to Investigate Microvascular Dysfunction in Trauma Conditions: A Review of the State‐of‐the‐Art. Advanced Biology, p.2400037.
- S. Lee, J. Ko, D. Park, S. R. Lee, M. Chung, Y. Lee, N. L. Jeon (2018) Lab Chip, 18(1), p.2686
- Apeldoorn, C., Safaei, S., Paton, J. and Maso Talou, G.D., 2023. Computational models for generating microvascular structures: Investigations beyond medical imaging resolution. WIREs Mechanisms of Disease, 15(1), p.e1579.
- Labilloy, G. (2024) Computational Methods for The Identification of Multidomain Signatures of Disease States (Doctoral dissertation, University of Cincinnati).
- Raut, S.S., Acharya, S., Deolikar, V. and Mahajan, S. (2024) Navigating the frontier: emerging techniques for detecting microvascular complications in type 2 diabetes mellitus: a comprehensive review. Cureus, 16(1).
- Duranteau, J., De Backer, D., Donadello, K., Shapiro, N.I., Hutchings, S.D., Rovas, A., Legrand, M., Harrois, A. and Ince, C., 2023. The future of intensive care: the study of the microcirculation will help to guide our therapies. Critical Care, 27(1), p.190.
- Park, S., Sharma, H., Kim, W., Gwon, Y., Kim, H., Choung, Y.H. and Kim, J., (2023). Microengineered organoids: reconstituting organ-level functions in vitro. Organoid, 3.
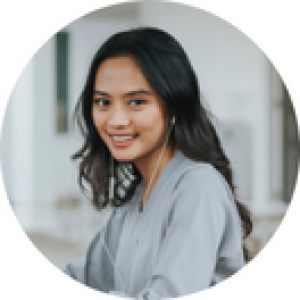
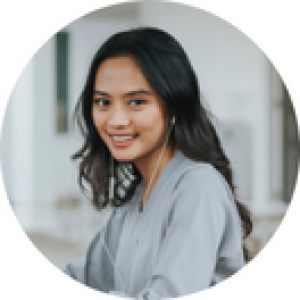