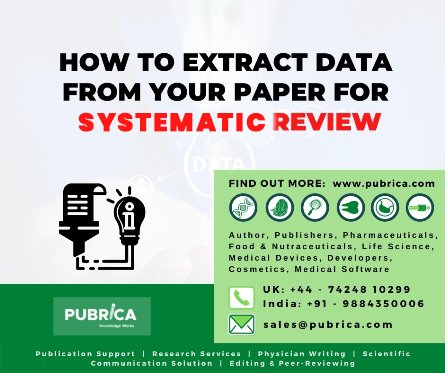
How to extract data from your paper for systematic review
August 16, 2021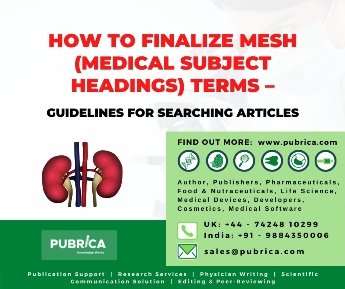
How to Finalize MeSH (Medical Subject Headings) terms – Guidelines for Searching Articles
August 23, 2021Cancer is one of the leading causes of mortality globally, and many research studies have focused in the last decade on developing novel therapeutics to lessen the negative effects of existing treatments. Tumors become extremely heterogeneous as cancer progresses, resulting in a mixed population of cells with varying molecular characteristics and therapeutic responses.This variability may be seen at both a geographic and temporal level, and it is a significant element in the establishment of resistance phenotypes, which is aided by a selection pressure applied during drug administration[1]. Typically, cancer is treated as a single, worldwide disease, and tumors are viewed as a group of cells.. As a result, a thorough knowledge of these complicated events is critical for developing accurate and effective treatments. Nanomedicine provides a diverse platform of biocompatible and biodegradable technologies for delivering traditional chemotherapeutic medicines in vivo, boosting their bioavailability and concentration surrounding tumor tissues, as well as their release profile. Nanoparticles can be used for a variety of purposes, from diagnostic to therapy[2].
Natural antioxidants in cancer therapy
Exogenous assaults to the human body, such as ultraviolet (UV) rays, air pollution, and tobacco smoke, cause the creation of reactive species, particularly oxidants and free radicals, which are responsible for the start of many diseases, including cancer. These molecules can be formed as a result of drug delivery in the clinic, but they can also be formed spontaneously inside our cells and tissues by mitochondria and peroxisomes, as well as macrophage metabolism, during normal physiological aerobic activities. DNA (genetic changes, DNA double strand breaks, and chromosomal abnormalities) and other bio-macromolecules, such as lipids (membrane peroxidation and necrosis) and proteins (substantially affecting the regulation of transcription factors and, as a result, of important metabolic pathways) can be damaged by oxidative stress and radical oxygen species [3].
Targeted therapy and immunotherapy
The low specificity of chemotherapeutic medicines for cancer cells is one of the key difficulties with conventional cancer treatments. In fact, most medications have severe side effects because they act on both healthy and sick organs.. Clinical Researchers are working hard to figure out how to target only the targeted site. Because of their enhanced permeability and retention effect (EPR), nanoparticles have sparked a lot of attention because of their tendency to aggregate more in tumor tissues. The small size of nanoparticles, as well as the leaky vasculature and poor lymphatic drainage of neoplastic tissues, are used in this passive targeting method[4]. Passive targeting, on the other hand, is difficult to regulate and can result in multidrug resistance (MDR)[5]. Active targeting, on the other hand, improves tumor cell uptake by targeting specific receptors that are overexpressed on the cells[6]. Nanoparticles, for example, can be functionalized with ligands that attach to specific cells or subcellular locations in an unambiguous manner[7] .
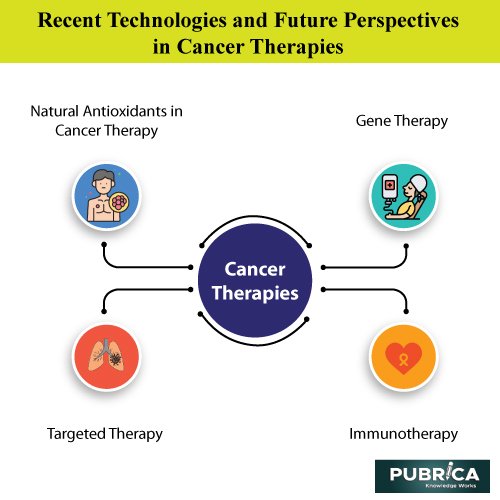
Gene therapy for cancer treatment
Gene therapy entails inserting a healthy copy of a faulty gene into the genome in order to treat a specific condition[8]. A retroviral vector was used to transfer the adenosine deaminase (ADA) gene to T-cells in patients with severe combined immunodeficiency (SCID) for the first time in 1990. Further study revealed that gene therapy might be used to cure a variety of human uncommon and chronic diseases, as well as, most significantly, cancer.. Approximately 2,900 gene therapy clinical trials are now underway, with cancer accounting for 66.6 percent of them[9]. Different strategies are under evaluation for cancer gene therapy: 1) targeted silencing of oncogenes, 2) expression of pro-apoptotic and chemo-sensitising genes 3) expression of genes able to solicit specific antitumour immune responses and 4) expression of wild type tumour suppressor genes.
Thermal ablation and magnetic hyperthermia
The term “thermal ablation of tumors” refers to a set of procedures that use heat (hyperthermia) or cold (hypothermia) to eliminate cancerous tissues[10]. Cell necrosis is reported to occur at temperatures as low as -40°C and as high as 60°C.. Cell necrosis is reported to occur at temperatures as low as -40°C and as high as 60°C.Furthermore, cancer cells have been demonstrated to be more susceptible to high temperatures than healthy cells.
The production of ice crystals upon cooling causes hypothermic ablation, which destroys cell membranes and eventually kills cells.The chosen cooling agent is argon gas, which may cool surrounding tissues to -160°C.Also, because nitrogen has a higher heat capacity than argon, gases at their critical point can be used. However, the technology for controlling and directing them is still in its infancy[11].
Radiomics and pathomics
The term “radiomics” refers to the high-throughput quantification of tumor features based on medical image analysis [12]. Pathomics, on the other hand, is dependent on the creation and analysis of high-resolution tissue images[13]. Many clinical research projects are focusing on the development of novel image processing techniques in order to extrapolate information through quantification and disease classification[14].To detect disease phenotypes, flexible databases are necessary to accommodate large amounts of data from gene expression, histology, 3D tissue reconstruction (MRI), and metabolic characteristics (positron emission tomography, PET)[15].
Conclusion and future prospective
In recent years, cancer research has made significant progress toward more effective, precise, and less intrusive cancer treatments.While nanomedicine in combination with targeted therapy improved the biodistribution of new or already tested chemotherapeutic agents around the specific tissue to be treated, additional techniques, such as gene therapy, siRNA delivery, immunotherapy, and antioxidant compounds, provide cancer patients with new options.Thermal ablation and magnetic hyperthermia, on the other hand, are potential alternatives to tumor resection.Finally, radiomics and pathomics techniques aid in the handling of large data sets generated by cancer patients in order to enhance prognosis and outcomes.
References
[1] I. Dagogo-Jack and A. T. Shaw, “Tumour heterogeneity and resistance to cancer therapies,” Nat. Rev. Clin. Oncol., vol. 15, no. 2, pp. 81–94, Feb. 2018, doi: 10.1038/nrclinonc.2017.166.
[2] C. Martinelli, C. Pucci, and G. Ciofani, “Nanostructured carriers as innovative tools for cancer diagnosis and therapy,” APL Bioeng., vol. 3, no. 1, p. 011502, Mar. 2019, doi: 10.1063/1.5079943.
[3] B. Halliwell, “Oxidative stress and cancer: have we moved forward?,” Biochem. J., vol. 401, no. 1, pp. 1–11, Jan. 2007, doi: 10.1042/BJ20061131.
[4] R. Bazak, M. Houri, S. El Achy, S. Kamel, and T. Refaat, “Cancer active targeting by nanoparticles: a comprehensive review of literature,” J. Cancer Res. Clin. Oncol., vol. 141, no. 5, pp. 769–784, May 2015, doi: 10.1007/s00432-014-1767-3.
[5] S. Barua and S. Mitragotri, “Challenges associated with penetration of nanoparticles across cell and tissue barriers: A review of current status and future prospects,” Nano Today, vol. 9, no. 2, pp. 223–243, Apr. 2014, doi: 10.1016/j.nantod.2014.04.008.
[6] S. Xu, B. Z. Olenyuk, C. T. Okamoto, and S. F. Hamm-Alvarez, “Targeting receptor-mediated endocytotic pathways with nanoparticles: Rationale and advances,” Adv. Drug Deliv. Rev., vol. 65, no. 1, pp. 121–138, Jan. 2013, doi: 10.1016/j.addr.2012.09.041.
[7] C. Pucci, C. Martinelli, and G. Ciofani, “Innovative approaches for cancer treatment: current perspectives and new challenges,” Ecancermedicalscience, vol. 13, Sep. 2019, doi: 10.3332/ecancer.2019.961.
[8] T. Friedmann, “A brief history of gene therapy,” Nat. Genet., vol. 2, no. 2, pp. 93–98, Oct. 1992, doi: 10.1038/ng1092-93.
[9] S. L. Ginn, A. K. Amaya, I. E. Alexander, M. Edelstein, and M. R. Abedi, “Gene therapy clinical trials worldwide to 2017: An update,” J. Gene Med., vol. 20, no. 5, p. e3015, May 2018, doi: 10.1002/jgm.3015.
[10] H. J. W. L. Aerts, “The Potential of Radiomic-Based Phenotyping in Precision Medicine,” JAMA Oncol., vol. 2, no. 12, p. 1636, Dec. 2016, doi: 10.1001/jamaoncol.2016.2631.
[11] A. Hervault and N. T. K. Thanh, “Magnetic nanoparticle-based therapeutic agents for thermo-chemotherapy treatment of cancer,” Nanoscale, vol. 6, no. 20, pp. 11553–11573, 2014, doi: 10.1039/C4NR03482A.
[12] P. Lambin et al., “Radiomics: Extracting more information from medical images using advanced feature analysis,” Eur. J. Cancer, vol. 48, no. 4, pp. 441–446, Mar. 2012, doi: 10.1016/j.ejca.2011.11.036.
[13] J. Kong et al., “Machine-Based Morphologic Analysis of Glioblastoma Using Whole-Slide Pathology Images Uncovers Clinically Relevant Molecular Correlates,” PLoS One, vol. 8, no. 11, p. e81049, Nov. 2013, doi: 10.1371/journal.pone.0081049.
[14] L. P. Clarke, B. S. Croft, R. Nordstrom, H. Zhang, G. Kelloff, and J. Tatum, “Quantitative Imaging for Evaluation of Response to Cancer Therapy,” Transl. Oncol., vol. 2, no. 4, pp. 195–197, Dec. 2009, doi: 10.1593/tlo.09217. [15] D. Romo-Bucheli, A. Janowczyk, H. Gilmore, E. Romero, and A. Madabhushi, “Automated Tubule Nuclei Quantification and Correlation with Oncotype DX risk categories in ER+ Breast Cancer Whole Slide Images,” Sci. Rep., vol. 6, no. 1, p. 32706, Dec. 2016, doi: 10.1038/srep32706.